The controversy over how we smell says as much about science as it does about olfaction.
A fragrant object lies before you—say, a flower, some stinky cheese, or a smelly sock. Molecules break off from the object, evaporating into the air and floating towards your face. A sniff pulls the molecules into your nostril, where they travel through your nasal cavity towards your olfactory neurons, which extend out through holes in your skull into the mucus layer of your nose. The molecules activate receptors studding the outside layer of individual neurons, which send a message to your brain that something smells.
Humans have around 350 types of receptors on 40 million neurons, which in combination allow us to distinguish more than a trillion different odors. Despite this incredible power of olfaction, the human sense of smell is often neglected, considered a holdover from our animal past or a source of unseemly sensations. It doesn’t help that odors are often literally beneath us; the evolutionary argument goes that when early humans started walking upright, our nose got farther away from the smells on the ground, decreasing the relative importance of olfaction for getting around. Evidence contradicting this story came from a 2006 study which recruited undergraduate students to get on all fours and track the scent of chocolate oil dripped on grass. They were remarkably good at it, showing that our “bad” sense of smell isn’t biologically determined—it’s just that we’re out of practice.
Despite our olfactory ignorance and several millennia worth of art historians looking down their noses at smell (Plato famously said that “beauty is the pleasant which comes through the senses of hearing and sight”), fragrance is a multibillion dollar industry, a growing part of the art world, and a fascinating area of basic science. It is also home to one of the biggest scientific controversies of our time: How do scent receptors work? That this fundamental question is a matter of debate highlights the uncertainties that attend our sense of smell and in the nature of scientific knowledge production itself.
We know a lot about how a smell signal travels from an activated receptor on a neuron to the brain. This pathway has been worked out in painstaking detail, with cascades of cellular switches and neural action potentials that signal our brain. Olfactory receptors have been mapped in the olfactory bulbs of flies and rats. They have been cloned into yeast, giving the microorganism a “human” sense of smell. Insect olfactory receptors have even been linked up with electronics, creating smelling cyborg robots that can turn their heads toward smells.
But how does a smelly molecule activate its receptor in the first place? There is still no satisfactory answer to this question despite nearly a century of research. Before the 1920s, many believed that the sense of smell worked at a distance, and that physical interactions between the nose and the molecules being smelled weren’t necessary. Now, the most widely held theory is that the interaction between a molecule and a receptor is like a physical lock and key—the shape of the molecule fits precisely into a hole in the receptor, which triggers the transmission of a smell signal to the brain.
While this theory has mostly held for decades and has been used by fragrance labs and olfaction researchers trying to predict smells based on shape, there are many molecular shapes and scents that don’t quite fit. There are molecules with very different shapes but very similar smells, like the 75 different molecules that all smell like the “bitter almond” of cyanide, and there are molecules that have very similar shapes but very different smells. The molecules below differ by only one atom—sulfur or oxygen—and are chemically very similar. But the molecule on the left, pinanethiol, smells, according to smell expert Luca Turin, like “a grapefruit from Hell” and the molecule on the right, pinanol, smells like pine needles.
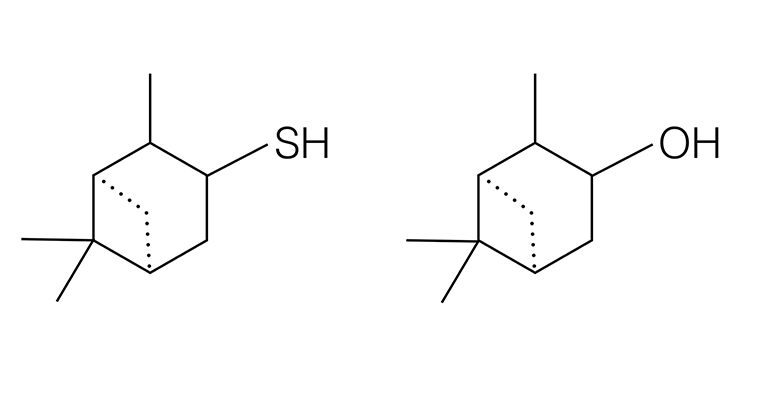
Sulfur and oxygen are in the same column of the periodic table, differing in mass but sharing some chemical attributes. The same smell-change phenomenon happens with other atomic replacements in the same column of the periodic table. Replace a single carbon atom with silicon in benzyldimethylmethoxy-methane and you can transform the smell from “camphor-radish like” to “flower-honey like with a minty component.” Bond two of the silicon versions to each other into bis(benzyl)tetramethyldisiloxane and you get a very weak flowerlike smell. Replace the silicon in that molecule with germanium and you get a relatively strong “almond-soapy like” odor.
Besides shape, what might explain these striking differences in smell? After World War I, the chemist Malcolm Dyson was experimenting with mustard oils, adding atoms to different spots on their molecules and smelling the results. At certain positions in the molecule, the extra atoms suddenly changed the smell from mustard to anise. Going down a column of the periodic table, the heavier the atom he added, the stronger the anise smell. Dyson speculated that perhaps there was a molecular vibration that caused the change in smell. Heavier atoms would vibrate at a different frequency than lighter ones. Could it be that the nose isn’t detecting the shape of the molecule, but instead what Dyson called the “osmic frequency” of the molecules?
Everything is constantly vibrating. Atoms jiggle and bounce, animated by thermal energy. The bonds between atoms can bend and vibrate, resonating like plucked strings tuned to the particular strength of the chemical interaction. Every molecule creates a spectrum of vibrations that reflect the combination of the vibrations of all the individual bonds that make up the molecule.
For Dyson, the idea that molecular vibrations might underlie our sense of smell suggested a tantalizing symmetry with our other senses. The rods and cones in our retinas respond to the vibrating wavelengths of light; the hair cells in our ear activate in response to the frequencies of sound waves vibrating in the air. Is smell also a vibrational sense?
Though the theory was compelling, Dyson didn’t have a clue of how the nose could actually detect the vibrations. Chemists identify molecules by blasting them with infrared light and detecting their resonant frequencies with specialized equipment, but the olfactory receptors were surely not shooting infrared light into the nose. Without a clear mechanism, the vibration theory languished for decades, periodically reappearing in the literature on olfaction only to disappear again under an avalanche of research papers about shape-based theories.
In the 1990s, Luca Turin, an academic biophysicist and an amateur perfume critic, was studying how electrons could flow through proteins. In his book The Secret of Scent, he describes how he eventually stumbled his way to the forefront of what became a scientific controversy over the nature of olfaction. “Many big decisions in our lives,” he explains, “are guided by aesthetic, even sentimental reasons”; his life-long love for perfume led him to make the leap between the behavior of electrons in his arcane biophysics experiments and the behavior of electrons in smell receptors. To Turin, this connection suggested a mechanism for vibration-sensing in the nose, which could revive Dyson’s theory of osmic frequencies.
In a 1996 paper, Turin proposed his version of the vibration theory of olfaction. His theory goes like this: each kind of olfactory receptor recognizes a specific shape and is also tuned to a particular vibrational frequency. Once the shape of the molecule fits in the receptor (like the “lock and key” mechanism of the shape theory), then the vibrational frequency of the molecule has to match the receptor’s frequency. If it’s a match, an electron can tunnel—a quantum jump where the electron is transported from one place to another without ever actually being anywhere in between—across the receptor and activate the neuron. A 2006 paper by UK physicists termed this the “swipe card model.” Their mathematical analysis of Turin’s model led them to conclude that “there are no physics-based objections.”
But that doesn’t mean there weren’t scientific objections. Turin first submitted his paper to the journal Nature, believing his theory to be of general interest to the scientific community and that therefore it shouldn’t be published in a specialized olfaction journal. The paper was eventually rejected after a year of back-and-forths with the journal editors, and eventually published in the journal Chemical Senses. Most papers submitted to Nature get rejected, but in The Secret of Scent, Turin argues that his paper was too interdisciplinary for Nature’s peer reviewers, who are experts in single disciplines. They must have “hated not being able to judge the rest and therefore assumed everything else must be worth hating too.” Not stopping there, later in the book Turin goes right for the scientific jugular: “scientists can be reluctant to let mere facts get in the way of well-established opinion.”
There are as many complaints about scientific rigidity as there are scientists who fancy themselves independent thinkers, but Turin is unique even amongst the self-proclaimed mavericks. He writes in support of anecdotal evidence, he cites the anarchist philosopher of science Paul Feyerabend’s polemical book Against Method, and he does it all in surprisingly public ways. It’s Turin’s willingness to make big claims in venues besides peer-reviewed articles that seems to have ruffled the most establishment feathers. A television documentary about his work came out around the same time as his Chemical Senses paper, and a book by Chandler Burr called The Emperor of Scent came out a few years later, elevating Turin to the status of a scientific martyr, shunned for his audacity to speak out publicly about the limitations of the shape-based theory of smell.
The identity of an element is defined by the number of protons in its nucleus. Add or remove some electrons and the element is the same, just with an ionic charge. Add or remove neutrons from the nucleus and it’s an isotope: same element, different mass. Stable isotopes (the unstable ones are radioactive) can be used to make molecules with the same shape but with different masses, and therefore different vibrational frequencies. “Heavy” hydrogen—called deuterium—has two neutrons instead of one, and is a stable isotope that can add extra mass to molecules. In Turin’s 1996 paper, he claims that “heavy” acetophenone made by replacing each hydrogen atom with deuterium is “fruitier and less toluene-like” and with a stronger bitter almond character than normal acetophenone.
A 2004 study published in Nature Neuroscience tried to refute this claim. The Rockefeller olfactory neurogeneticists Andreas Keller and Leslie Vosshall asked 36 non-expert smellers to compare normal and heavy acetophenone and found that people were unable to detect a difference. To the editors of Nature Neuroscience, the case was closed. An editorial supplement to the paper said that Turin’s theory, “though provocative, has almost no credence in scientific circles. The only reason for the authors to do the study, or for Nature Neuroscience to publish it, is the extraordinary—and inappropriate—degree of publicity that the theory has received from uncritical journalists.”
But that wasn’t the end of Turin’s story. Working from the Fleming Center for Biomedical Sciences in Greece, Turin published a paper showing that fruit flies could distinguish between light and heavy acetophenone in 2011. Then, in 2013 he and his team went back to human subjects. They verified Keller and Vosshall’s finding that humans can’t in fact detect the perhaps subtle difference between light and heavy acetophenone, speculating that it’s perhaps the molecule’s small size that makes the relative difference in vibration between the light and heavy molecules undetectable. To test this hypothesis they created “heavy musk”—the much larger musk molecule would have a bigger difference in its vibration spectrum when all of its 28 hydrogen atoms (compared to acetophenone’s 8) were replaced with deuterium. With the larger molecule, the human subjects could suddenly tell the difference. The deuterated musks smelled like musk plus something nutty, roasted, and burnt. These papers renewed public interest in the vibration theory, and dozens of news articles came out about the controversy, with titles like “‘Quantum Smell’ Idea Gains Ground.”
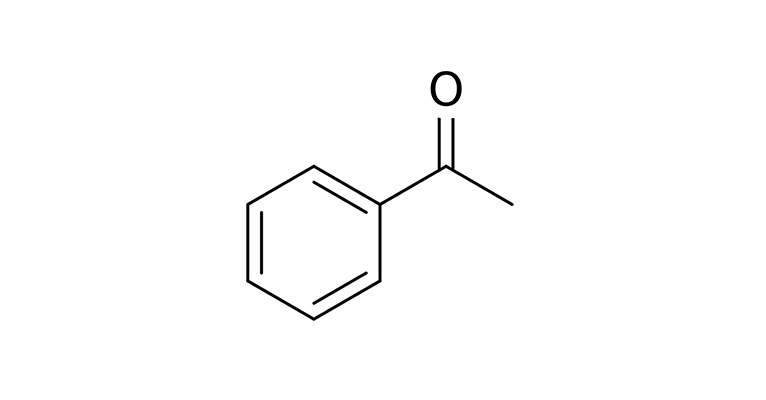
Many scientists remain unconvinced. Though Turin does have a hypothesis about the mechanism, he still doesn’t have proof that is satisfying to the wider olfaction community, and the controversy remains surprisingly bitter, as much about the very nature of scientific progress overall as it is about this particular theory. In an interview with BBC News following the publication of Turin’s 2013 paper, Leslie Vosshall was quoted saying, “I like to think of the vibration theory of olfaction and its proponents as unicorns. The rest of us studying olfaction are horses. The problem is that proving that a unicorn exists or does not exist is impossible. This debate on the vibration theory or the existence of unicorns will never end, but the very important underlying question of why things smell the way they do will continue to be answered by the horses among us.”
There is a persistent myth that science is done by unicorns, the lone genius toiling in his—it’s almost always his—laboratory, seeing what no one else can see. Luca Turin fulfills this romantic ideal. He is isolated, he is brash, and he is condemned by the establishment. He is the lonely visionary still fighting for the vibration theory, a decade after the horses have moved on.
Indeed, it is perhaps Turin’s outsider status that may explain both his public popularity and the hostility of the community of scientists who study smell. In “How to be Antiscientific,” Steven Shapin, a historian and philosopher of science, describes how science closes ranks against perceived threats from the outside, but often the harshest criticism of a field or of science in general comes from the scientists themselves. “We are now,” Shapin writes, “it seems, on the familiar terrain of everyday life: members of a family are permitted to say things about family affairs that outsiders are not allowed to say.” Respected scientists like Niels Bohr, Erwin Chargaff, E.O. Wilson, and Albert Einstein can get away with statements like, “there is no such thing as the Scientific Method,” whereas when philosophers like Shapin or Feyerabend say the same thing, they are intruders threatening the sanctity of science. Leslie Vosshall and her horses can point out the failures of the shape-based olfactory theories; Luca Turin, with his peculiar mix of biophysics and bravado, can’t.
Nevertheless, Turin’s unicorn of a theory is compelling, to many scientists and non-scientists alike, even if publicly supporting it may still be “scientific suicide.” The idea that we are all walking around with quantum spectroscopes in our faces is fascinating, even if we’re still trying to understand it through anecdotes and unreliable noses rather than hard biochemical data. Quantum smelling belongs to a strange class of scientific ideas, which Turin typologizes in The Secret of Scent: there are ideas that are of interest only to the specialists who study them, there are theories (related to cancer, for example) that are of widespread interest, and then there is a peculiar third category of scientific hypotheses: “things which are interesting even if not true.”
Even more than the idea of quantum smelling, Turin’s story is interesting for the way that it exposes the metascientific work that underlies scientific change. It’s rare for insider scientific conversations and controversies to become public before they become facts. We’re usually taught a version of science and its history that is a long list of such facts, without any of the personalities, the arguments, and the uncertainties that go into the making of science. Turin has let us inside the world of perfumery and olfaction, showing us something potentially remarkable about our biology at the atomic scale and the practice of biology at the human scale. That’s interesting whether or not his vibration theory is true.